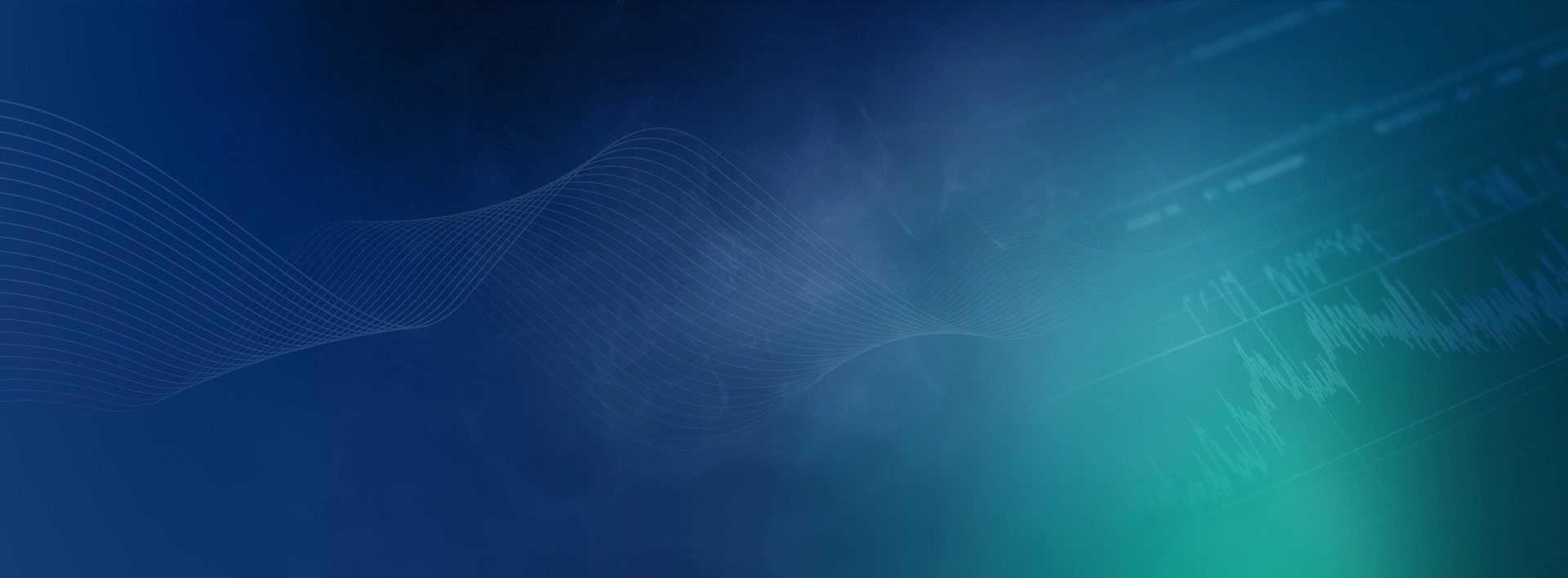
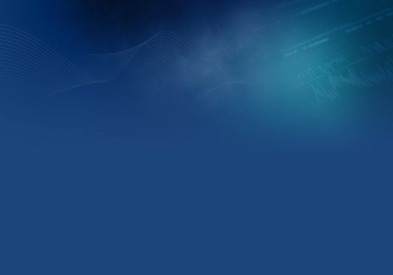
OSA: A Serious Sleep-
Related Breathing Disease
Obstructive sleep apnea (OSA) is a serious chronic sleep-related breathing disease where the upper airway repeatedly collapses during sleep, causing intermittent oxygen deprivation.
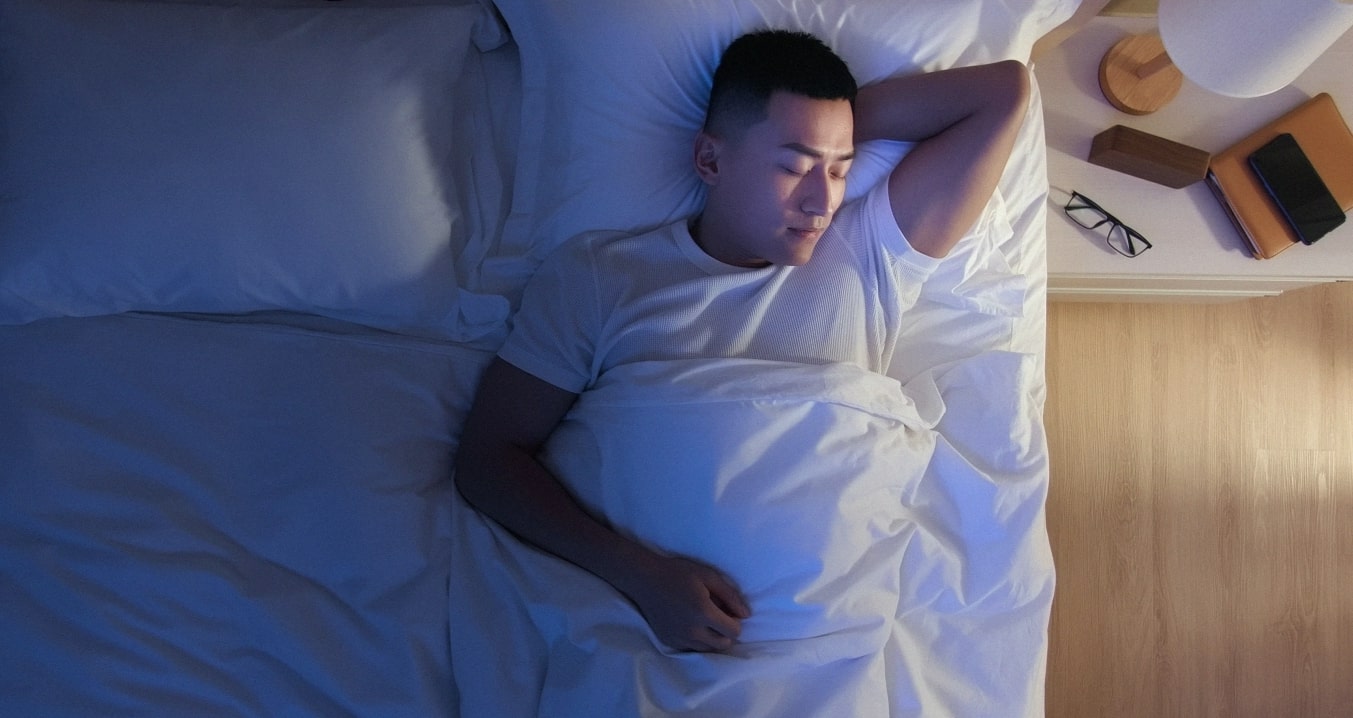
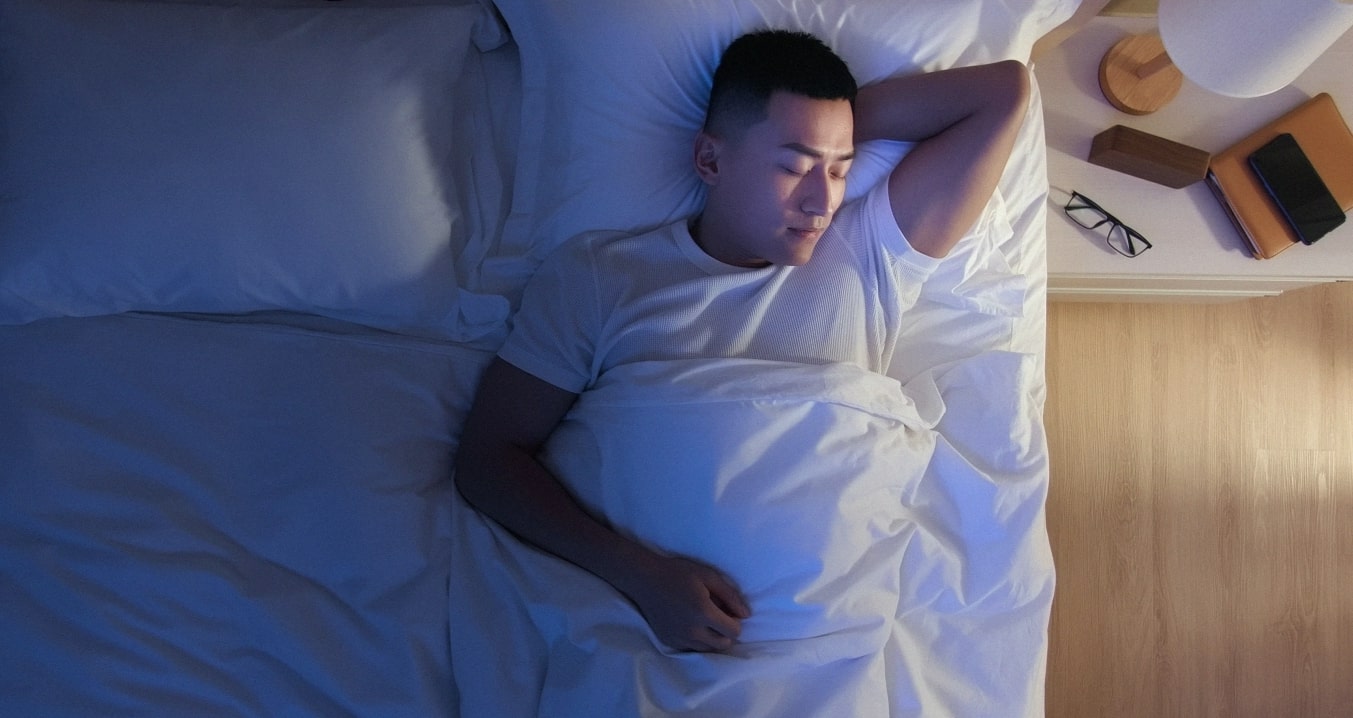
Every night, more than
54
million Americans
with OSA stop breathing, exposing them to serious, long-term health risks.1
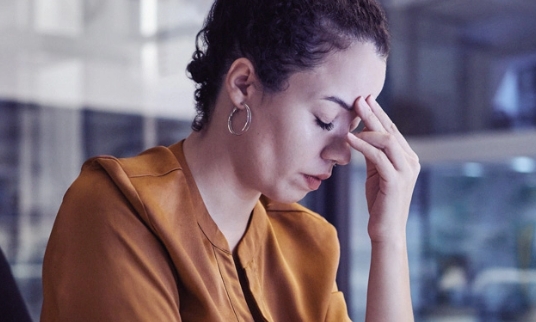
The Burden of
Oxygen Deprivation in OSA
An individual with OSA can experience hundreds of sleep apnea events in a single night—with breathing interruptions as often as once every minute.2
Each sleep apnea event reduces blood oxygen levels (oxygenation) and deprives cells of the energy they need to perform vital functions. The lack of oxygen shocks the body into survival mode and can lead to spikes in blood pressure, fluctuations in heart rate, and cyclical stress.2
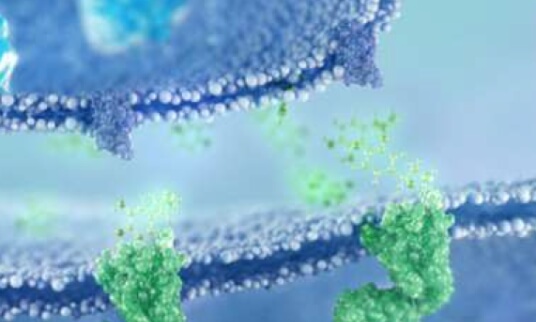
The Need for a
Neuromuscular Approach to Improve Oxygenation
Today’s standard of care falls short of meeting the needs of the community, leaving many people without an effective treatment for their OSA.
In a disease characterized by complex and invasive treatment options, AD109 could be the first pharmacological treatment to improve oxygenation during sleep by directly addressing the underlying neuromuscular cause of upper airway collapse in people with OSA. If approved, AD109 could simplify the treatment of OSA as an oral pill—improving the health and well-being for people living with sleep-related breathing diseases.
- NIH-funded study explains link to increased cardiovascular risks for people with obstructive sleep apnea. NIH.gov. Published July 26, 2023. Accessed September 12, 2024. https://www.nih.gov/news-events/news- releases/nih-funded-study-explains-link- increased-cardiovascular-risks-people- obstructive-sleep-apnea
- The hypoxic burden of sleep apnoea predicts cardiovascular disease-related mortality: the Osteoporotic Fractures in Men Study and the Sleep Heart Health Study. Azarbarzin A et al. Eur Heart J. 2019;40(14):1149-1157.
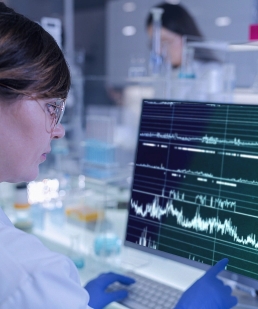
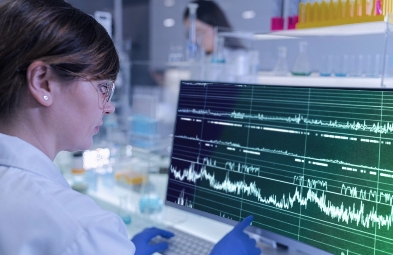
See How We’re Taking On Sleep-Related Breathing Diseases
Explore our active clinical programs and partnerships.
- NIH-funded study explains link to increased cardiovascular risks for people with obstructive sleep apnea. NIH.gov. Published July 26, 2023. Accessed September 12, 2024. https://www.nih.gov/news-events/news- releases/nih-funded-study-explains-link- increased-cardiovascular-risks-people- obstructive-sleep-apnea
- The hypoxic burden of sleep apnoea predicts cardiovascular disease-related mortality: the Osteoporotic Fractures in Men Study and the Sleep Heart Health Study. Azarbarzin A et al. Eur Heart J. 2019;40(14):1149-1157.
AD109 Data in OSA
Read More